Introduction
Viruses are obligate parasites that require hosts for their replication. As they replicate within their hosts, viruses mutate and develop quasispecies, selected for the next generation. While most viral mutations are deleterious, a sufficiently large population of variants can confer some with the capacity for interspecies transmission or the evasion of virus-specific immune responses (Acevedo et al., 2014; McCrone & Lauring, 2018).
DNA viruses typically have mutation rates ranging from 10−8 to 10−6 substitutions per nucleotide site per cell infection (s/n/c), while RNA viruses exhibit higher mutation rates between 10−6 and 10−4 s/n/c (Peck & Lauring, 2018). High mutation rates allow viruses to adapt and survive in specific host environments through selected mutations. The pressures that select for the next generation of viruses and their mutants are varied and include factors such as antiviral agents, immune responses, and host switching, all of which influence viral fitness within the environment (Wargo & Kurath, 2012).
Host switching is relatively rare, as viruses typically infect a narrow range of host species, though this spectrum varies among different viruses (Rothenburg & Brennan, 2020). Nevertheless, as observed with the recently emergent severe acute respiratory syndrome coronavirus 2 (SARS-CoV-2), which is linked to the intermediate horseshoe bat (Rhinolophus affinis) and the Malayan pangolin (Manis javanica), viruses can switch hosts, potentially leading to pandemics in new host species (Lam et al., 2020; Zhou et al., 2020). Consequently, studying viral mutations across different host species can offer insights into viral evolution and help prepare for future pandemics.
In this study, we used the Shaan virus isolate (B16-40), a novel species from the family Paramyxoviridae originating from bats (Noh et al., 2018). Considering that recent viruses affecting humans have originated from bats, studying a novel paramyxovirus from bats, previously unknown to infect humans, is crucial for understanding its mutation patterns and potential to infect humans. In this context, both high- and low-passaged Shaan viruses in MARC-145 cell lines derived from African green monkey kidney (Kim et al., 1993) were introduced to A549, HEK-293, and HRT-18 cell lines (human origin). These cell lines served as models for different host species. Utilizing population-based analysis, which is important for studying viral evolution due to the quasispecies nature of viruses (Geoghegan & Holmes, 2018), we examined mutations in the high- and low-passaged Shaan viruses from both the original and new host environments using high-throughput sequencing in triplicate.
Materials and Methods
Cells and viruses
MARC-145 cell lines, derived from the kidney of the African green monkey, were cultured in Dulbecco’s Modified Eagle Medium (cat. no. 11995; Gibco, NY, USA), supplemented with antibiotic-antimycotic (100 IU/mL penicillin, 100 μg/mL streptomycin, and 0.25 μg/mL amphotericin B) (cat. no. 15240; Gibco, NY, USA) and 10% fetal bovine serum (cat. no. SV30207; Hyclone, NY, USA). Human-origin HEK-293, A549, and HRT-18 cell lines from kidney, lung, and colon, respectively, were cultured in MEM (cat. no. 10370; Gibco, NY, USA) and RPMI1640 (cat. no. A10491; Gibco, NY, USA) media, supplemented with 10% fetal bovine serum and antibiotic-antimycotic. These cells were maintained at a constant 37°C and 5% CO2 in an incubator.
The Shaan virus isolate (B16-40) (GenBank accession no. MG230624.1) was propagated in MARC-145 cells. For amplification, 1 × 105/mL of MARC-145 cells were seeded in tissue culture flasks using Dulbecco’s Modified Eagle Medium supplemented with 5% fetal bovine serum and incubated overnight. Following this, a MARC-145 cell monolayer was infected with the Shaan virus (B16-40) at a multiplicity of infection (MOI) of 0.1, with the cytopathic effect observed in the infected cells five days post-inoculation. The virus, harvested by freeze-thawing the infected cells, was then used to infect a fresh monolayer of MARC-145 cells for further passages.
Preparation of low-passaged and high-passaged Shaan virus in MARC-145 cells
The original isolate of Shaan virus (B16-40) (GenBank accession no. MG230624.1) was passaged in MARC-145 cells, as depicted in Fig. 1. Low-passaged virus was obtained by serially passing the virus four times in MARC-145 cells, while the high-passaged virus was derived from 44 serial passages in the same cell line. Viral titers of both, low- and high-passaged viruses, were quantified using the tissue culture infectious dose 50 (TCID50) method as described by the Reed-Muench formula.
Host switching of low-passaged and high-passaged Shaan virus
The low-passaged (p4) and high-passaged (p44) Shaan virus samples from MARC-145 cells were inoculated in triplicate into various host cells, specifically HEK-293, A549, and HRT-18 cell lines, originating from human kidney, lung, and colon respectively, as indicated in Fig. 1. Each virus was introduced into these cell lines with a multiplicity of infection (MOI) of 0.5 and allowed to infect for 2 hours. Post-infection, cells were washed with DPBS (pH 7.4) (cat. no. LB001-02; Welgene Inc., Daegu, Korea) and were further incubated with the respective maintenance medium for 24 hours, following protocols established by Lim et al., (2023). Virus harvesting was carried out by freeze-thawing the infected cells, and the collected viruses were then utilized for further quantification and sequencing analyses.
Viral RNA quantification
Total RNA was extracted from harvested samples using the RNeasy Mini Kit (Qiagen) according to the manufacturer’s instructions. Viral RNA was quantified using two real-time RT-PCR methods that targeted total viral RNAs and the viral antigenome. For total viral RNA quantification, cDNAs were synthesized using random hexamers and tested through real-time PCR with a Shaan virus detection primer, which targeted the small hydrophobic (SH) protein region (Supplementary Table 5). Antigenome quantification was performed using a primer designed specifically for the intergenic region between the nucleocapsid and phosphoprotein, suitable for reverse transcription. This antigenome was quantified in real-time PCR using an antigenome-specific primer (Supplementary Table 5). Viral RNA and antigenome levels were normalized to the monkey (for MARC-145 cells) and human GAPDH genes as controls (Das et al., 2000; Shi et al., 2016). Cell lysates from all Shaan virus-infected cells were inoculated into MARC-145 cells, and infectious virion titers were quantified as tissue culture infectious dose 50 (TCID50) using the Reed-Muench method.
Sequencing and analysis
RNA was extracted from infected cells harboring low-passaged (p4) and high-passaged (p44) Shaan virus using TRIzol LS Reagent (Thermo Fisher Scientific Inc.), according to the manufacturer’s manual. RNA samples were analyzed by Macrogen, Inc. (Seoul, Republic of Korea) for high-throughput sequencing and quality assessment. Subsequently, total cDNA libraries, sourced from RNA that met quality standards, were prepared using the Illumina TruSeq Strand Total RNA LT kit according to the manufacturer’s instructions. The samples were sequenced on a NovaSeq 6,000 platform.
Data quality of the raw sequences was verified using FastQCv0.11.7. Subsequently, adapter sequences were excised using BBDuk adaptor and quality trimming version 38.84 (Bushnell, 2014). The sequences of the trimmed reads from both low- and high-passaged viruses were aligned to the Shaan virus sequence (GenBank accession no. MG230624.1) using the Geneious Prime Bowtie 2 plugin version 2.3.0 (Langmead & Salzberg, 2012). The expression levels of transcripts in cells infected with Shaan virus were quantified based on a reference annotation of Shaan virus (MG230624.1). Transcript abundance was calculated and normalized to the TPM value, considering read count, transcript length, and coverage depth. Three consensus genomic sequences obtained from Geneious Prime Bowtie 2 plugin version 2.3.0 (default setting) within the same group were aligned using the MAFFT v7.450 auto alignment algorithm (Katoh & Standley, 2013). The consensus genomic sequences of low- and high-passaged viruses served as reference sequences for mutation pattern and SNV analysis. The mean read depth of coverage for these genomic sequences is presented in Supplementary Table 1. Selected mutations were analyzed using the consensus genomic sequences of viruses passaged once in A549, HEK-293, and HRT-18 cells compared to Shaan virus in MARC-145 cells using Geneious software, with analysis of synonymous and non-synonymous amino acid substitutions also performed.
For SNV analysis, trimmed reads from both low-passaged and high-passaged viruses, passaged once in A549, HEK-293, and HRT-18 cells, were mapped to the consensus genomic sequences of the original viruses. Single nucleotide polymorphisms (SNPs) and INDELs were detected at minimum variant frequencies of 0.25 or 0.05, with a 10−6 maximum variant p-value and a 10−5 minimum strand-bias p-value (exceeding 65% bias) using the Geneious software workflow, “Map reads then find variations/SNPs”. The coverage and variant frequency data for the SNPs and INDELs across each experimental group are detailed in Supplementary Tables 2, 3, and 4. SNV results with coverage below 100× were omitted from the mutation analysis. Synonymous and non-synonymous amino acid substitutions at these SNVs were further examined.
Statistical analysis
All data in triplicate were subjected to a normality test using an unpaired t-test with multiple comparisons across groups. Statistical analyses were conducted using one-way ANOVA with Tukey’s multiple comparison test in GraphPad Prism version 9.0. Asterisks denote p-values as follows: *p < 0.05; **p < 0.01; ***p < 0.001; ****p < 0.0001; ns, not significant.
Results
Quantitative analysis of Shaan virus replication in HEK-293, A549, and HRT-18 cells
Low- and high-passage viruses were infected into HEK-293, A549, and HRT-18 cells. At 24 hours post-infection, viral replication was compared by measuring total viral RNA, antigenomes, and infectious virions (Fig. 2). Relative viral RNA quantities in HRT-18 cells were significantly higher than those in A549 and HEK-293 cells, regardless of the Shann virus inoculum and the virus passage level. Additionally, when antigenomes produced during viral replication in host cells were measured, the relative amount of antigenomes was significantly higher in HRT-18 cells than in A549 and HEK-293 cells. Infectious virions were measured using TCID50 in MARC-145 cells, where a significantly higher number of infectious virions was observed in HRT-18 cells infected with either low- or high-passage viruses.
Comparison of Shaan virus-specific transcripts in low- and high-passage virus-infected HEK-293, A549, and HRT-18 cells
Using high-throughput sequencing, a total of 24 sets of sequencing data were obtained, representing triplicate samples from either low- or high-passage Shaan virus, as well as those-infected HEK-293, A549, and HRT-18 cells, as documented in Supplementary Table 1. The total read counts varied from 50,285,454 to 76,298,278. The reference mapping of trimmed reads against a reference genome (GenBank accession no. MG230624.1) showed different coverage values. In HRT-18 cells, the mean read depth for mapping ranged from 2,014.9 to 2,387.8 in the low-passage Shaan virus-infected cells and from 2,177.2 to 2,384.0 in the high-passage Shaan virus-infected cells. Notably, in HEK-293 cells, the mean read depth ranged from 4,553.6 to 6,452.6 in the low-passage Shaan virus-infected cells, but only from 9.4 to 17.0 in the high-passage Shaan virus-infected cells. In A549 cells, mean read depths were 517.0-1,049.1 after low-passage virus infection and 305.0-802.1 following high-passage virus infection. The percentage rate of mapped reads with a Phred score > 30 ranged from 98.2% to 98.7% in all groups.
After reference mapping the sequenced reads, Shaan virus-specific transcripts were compared based on different viral genes. The mapped reads were normalized and expressed as transcripts per million (TPM) values (Fig. 3). Overall, transcript profiles were found to be similar to those of other paramyxoviruses and Shaan virus (Wignall-Fleming et al., 2019; Lim et al., 2023). No significant differences were observed in the TPM values of each gene between cells infected with low- and high-passaged Shaan virus. However, transcripts associated with the nucleocapsid (N) and matrix (M) genes varied among the host cells. Shaan virus-specific transcripts associated with the N gene were the highest, followed by those associated with the M gene in MARC-145, A549, and HEK-293 cells, regardless of the virus passage level. In HRT-18 cells, transcripts associated with the M gene were the highest, followed by those associated with the N gene.
Genomic differences between low- and high-passaged Shaan viruses in MARC-145 cells
Consensus genomic sequences of low- and high-passaged Shaan viruses were obtained from triplicate high-throughput sequencing, using the reference genome of the Shaan virus (GenBank accession no. MG230624.1) for mapping the trimmed reads. Six mutations were identified in the low-passaged Shaan virus (p4), and nine mutations in the high-passaged Shaan virus (p44) (Fig. 4). Three mutations in the hemaglutinin-neuraminidase (HN) gene, specifically non-synonymous substitutions C9,724T (H239Y in amino acids), C9,803T (A265V), and T10,484C (I492T), were consistently observed in both virus passages. Additionally, in the low-passaged viruses, non-synonymous substitutions A5,876T (K435N) in the fusion protein (F) gene and G6,676A (E129K) in the small hydrophobic protein (SH) gene were observed. The high-passaged virus exhibited additional non-synonymous substitutions T4,590A (F7A) in the F gene, C11,194T (S49F), and C13,936T (T964I) in the large protein (L) gene. The mutations found in the low- and high-passaged viruses were consistent across triplicate sequencing runs.
We also analyzed single nucleotide variants (SNVs) in low- and high-passaged viruses. The consensus genomic sequences we obtained were used as references for SNV analysis. SNVs found in low-passaged Shaan virus (p4) were more numerous than those in the high-passaged Shaan virus (p44) (Fig. 5). With a minimum variant frequency of 0.25, six SNVs across the F, SH, TM, HN, and L genes were identified in the low-passaged virus, compared to only one SNV in the high-passaged virus. The SNV in the high-passaged virus was characterized by the insertion of adenine (A) at an A-rich site, potentially due to a sequencing error. With a minimum variant frequency of 0.05, 15 SNVs were observed in the low-passaged virus, whereas seven SNVs were detected in the high-passaged virus.
Mutations found in infected low- and high-passaged Shaan viruses after one passage in A549, HRT-18, and HEK-293 cells
Consensus genomic sequences from low-passaged (p4) and high-passaged (p44) Shaan viruses in MARC-145 cells served as references to generate consensus genomic sequences of the viruses passaged once in A549, HRT-18, and HEK-293 cells. These consensus genomic sequences of the once-passaged viruses were compared with the original sequences of low- and high-passaged Shaan viruses. No noticeable selected mutations were found when low-passaged and high-passaged Shaan viruses were passaged once in A549 cells (Fig. 6). Y(C/T) 8,057T on the TM gene and A13,706R (A/G) on the L gene were observed at the SNV sites in the virus passaged in A549 cells following infection with low-passaged Shaan virus (p4); similarly, A4,590W (A/T) on the F gene was observed at the SNV sites in the virus passaged in A549 cells following infection with high-passaged Shaan virus (p44).
In the case of HEK-293 cells, no selected mutations were observed following one passage of the low-passaged Shaan virus (p4). As noted in Supplementary Table 1, the low quality of the mapped reads precluded the detection of selected mutations in the viruses following one passage of the high-passaged Shaan virus (p44) in HEK-293 cells.
Selected mutations occurred more frequently in viruses passaged once in HRT-18 cells. When low-passaged Shaan virus (p4) was passed once through HRT-18 cells, nine selected mutations were detected. Among these, T2,834C (I372T) and T2,852C (F378S) in the phosphoprotein (P) gene, T5,876A (N435K) in the F gene, A6,676G (K129E) in the SH gene, and C10,484T (T492I) in the HN gene were non-synonymous substitutions. In the case of the high-passaged Shaan virus (p44) passed once through HRT-18 cells, 12 selected mutations were detected. These included consistent mutations like T2,834C (I372T) and T2,852C (F378S) on the phosphoprotein (P) gene, and C10,484T (T492I) on the HN gene from the same cells infected with low-passaged virus. Additional non-synonymous substitutions such as A4,590T (I7F) on the F gene and T11,195C (F49S) and T13,940C (I964T) on the L gene were also observed.
Single nucleotide variants (SNVs) found in low- and high-passaged Shaan viruses after one passage in A549, HRT18, and HEK293 cells
We also analyzed the SNVs of the viruses that were passaged once in A549, HRT-18, and HEK-293 cells with low- and high-passaged Shaan viruses. The consensus genomic sequences obtained for mutation pattern analysis served as references for the SNV analysis of each group. With a minimum variant frequency of 0.05, we found no consistent SNVs between viruses after one passage in A549 cells with low- and high-passaged Shaan viruses (Fig. 7A), except for the 2,415A insertion on the P gene. As the insertion site was in a repeated A sequence, this might be a sequencing error. Although inconsistent SNVs were observed in one sample of three replicates in the low passage-virus-infected A549 cells, all the SNVs from the other low-passaged Shaan virus passaged once in A549 cells matched those found in the original low-passaged virus. In the high-passaged Shaan virus-infected group, out of a total of eight SNVs, five SNVs consistently appeared in the same positions as in the original high-passaged Shaan virus.
A similar pattern was observed in HEK-293 cells (Fig. 7B). Here, all the SNVs in the viruses from one passage of low-passaged Shaan virus in HEK-293 cells matched those in the original low-passaged virus. However, due to low coverage, we were unable to interpret the SNVs in high-passaged virus-infected HEK-293 cells.
Notably, SNVs found in the viruses after one passage in HRT-18 cells were relatively unique compared to those in the other groups (Fig. 7C). Among the eight SNVs found in the viruses from one passage of low-passaged Shaan virus, two SNVs were consistent with the original virus, while the others were HRT-18 cell-specific. Additionally, of the eight SNVs found in the viruses from one passage of the high-passaged Shaan virus, two were consistent with the original virus.
Discussion
Viruses continuously evolve in the infected host. High mutation rates allow viruses a chance to survive through the selected mutants in a given host environment. Certain host environments, such as antiviral drugs, host switching, and immune responses, act as selection factors for the next generation of viruses. Because mutations occur randomly (Luria & Delbrück, 1943; Murray, 2016), given the host environment, certain selected mutations from the random mutation pool may drive viral evolution. In this context, the serial passage of viruses in a particular cell line typically results in highly adapted viruses with changes in virulence and host specificity, which can be applied to the development of attenuated vaccine strains and animal models of human viruses (Song et al., 2007; Sanders et al., 2016; Li et al., 2017; Dinnon et al., 2020).
In this context, we investigated selected mutation patterns in a Shaan virus isolate by conducting host-switching experiments in MARC-145 cells (Noh et al., 2018). We prepared low-passaged (p4) and high-passaged (p44) Shaan viruses in MARC-145 cells to infect new hosts, specifically A549, HRT-18, and HEK-293 cells of human origin, and then conducted high-throughput sequencing for genetic analysis. As illustrated in Fig. 4, distinct mutations were identified between the low-passaged (p4) and high-passaged (p44) Shaan viruses in MARC-145 cells. In SNV analysis, using a minimum variant frequency of 0.25, six SNVs were detected in the F, SH, TM, HN, and L genes of the low-passaged virus, whereas only one SNV was identified in the high-passaged virus. Given that SNVs in the population are linked to selected mutations that drive evolution (Casci, 2010; Li et al., 2022), the high-passaged Shaan virus (p44) may exhibit better adaptation to MARC-145 cells than the low-passaged virus (p4). Subsequently, we compared the replication and genetic changes between the highly adapted and less adapted viruses in MARC-145 cells when introduced to new host cells (A549, HRT-18, and HEK-293 cells).
We observed replication of Shaan virus in three human cell lines. Given that its receptor was identified as alpha 2,3 sialic acids and it could trigger an innate immune response in HEK293 and A549 cells (Jang et al., 2022; Lim et al., 2023), replication of Shaan virus in these cell lines is expected. Although both low- and high-passaged viruses were able to replicate and produce infectious virions after one passage in A549, HRT-18, and HEK-293 cells, the high-throughput sequencing data for generating a consensus genomic sequence was insufficient in HEK-293 cells infected with high-passaged Shaan virus (Supplementary Table 1). As high-passaged Shaan virus (p44) was better adapted to MARC-145 cells than low-passaged Shaan virus (p4), it may not replicate efficiently in HEK-293 cells. However, when the viruses successfully infected new host cells, selected mutations were observed after one passage in the new host cells, regardless of whether they were A549, HRT-18, or HEK-293 cells. When low-passaged Shaan virus (p4) was passaged, no mutations were found in HEK-293 cells and two in A549 cells, while nine selected mutations were observed in HRT-18 cells, despite all host cells being of human origin. Although reliable consensus genomic sequences from high-passaged Shaan virus-infected HEK-293 cells were not available due to low mean read depth, distinct differences in the selected mutations were evident between the high-passaged Shaan virus-infected A549 and HRT-18 cells. Interestingly, although data were derived from triplicate experiments, the mutation patterns consistently occurred in the same host cells. These findings suggest a host cell-specific selection pattern for the further evolution of Shaan viruses within a single passage, which may be linked to cell type rather than cell origin.
In this study, HRT-18 cells may serve as a unique host for Shaan viruses. Regardless of whether the Shaan virus infections were low- or high-passaged, viruses that had passed through HRT-18 exhibited more unique selected mutations than those from other cells. Furthermore, Shaan virus replication in HRT-18 cells differed from that in other cell types. As depicted in Fig. 2, Shaan viruses passaged in HRT-18 cells demonstrated significantly enhanced replication properties and yielded higher quantities of infectious virions. Additionally, the level of Shaan virus-specific transcripts associated with the N gene was highest in MARC-145, A549, and HEK-293 cells, regardless of the infection’s nature, whether low- or high-passaged, and transcripts related to the M gene were most abundant in HRT-18 cells. Relative to other cells, HRT-18 cells infected with low- and high-passaged virus had higher counts of unique SNVs, with six unique SNVs each (Fig. 7). Given that SNPs in a population are linked to selected mutations for evolution (Casci, 2010; Li et al., 2022), it can be inferred that these unique SNVs found in viruses post HRT-18 cell passage may contribute to a greater diversity of Shaan virus variants in these cells. HRT-18 cells are a known colorectal adenocarcinoma cell line. A previous study reported increasing frequencies of novel cryptic SARS-CoV-2 lineages in feces-associated wastewater (Smyth et al., 2022). Thus, additional studies examining the relationship between intestinal infection-specific mutations and the emergence of future variant viruses are warranted.
In conclusion, the selected mutations of high-passaged (p44) and low-passaged Shaan virus (p4) after host switching from MARC-145 cells to A549, HRT-18, and HEK-293 cells differed from each other, even when the switched host cells were of the same human origin. Compared to the low-passaged virus, the high-passaged Shaan virus did not generate sufficient high-throughput sequencing data to produce a consensus genomic sequence in HEK-293 cells. However, when analyzing the others, mutation patterns were consistent across the same host cells in triplicate experiments, suggesting a host cell-specific selection pattern for the next generation of Shaan viruses. Among the cells in this study, HRT-18 cells, originating from colorectal adenocarcinoma, produced a more distinct mutation pattern, as well as unique SNVs, during both high- and low-passaged Shaan virus infections. Therefore, this study provides potential evidence for host-specific selective mutation patterns by cell type, as well as host cells, for Shaan virus evolution.
Acknowledgments
This research was supported by the Young Researcher Program through the National Research Foundation of Korea (NRF), which is funded by the Ministry of Science and ICT of Korea (NRF-2020R1C1C1010440), the Bio & Medical Technology Development Program through the NRF, funded by the Ministry of Science & ICT (2021M3E5E3083402), and the Basic Science Research Program through the NRF, funded by the Ministry of Education (2020R1A6A1A06046235).
Data Availability
The partial genome sequence of the bat paramyxovirus isolate Bat-ParaV/B16-40 has been deposited in GenBank with the accession number MG230624. The RNA-seq raw data are available in the Gene Expression Omnibus database under the GEO Series accession number GSE201344. Additionally, raw sequence reads can be accessed under the BioProject accession number PRJNA830904 and the SRA accession number SRP371774.
Author Contributions
Conceptualization: Ji Yeong Noh, Hye Kwon Kim; Data curation: Ji Yeong Noh; Formal analysis: Ji Yeong Noh, Hye Kwon Kim; Funding acquisition: Hye Kwon Kim; Investigation: Ji Yeong Noh; Methodology: Ji Yeong Noh; Project administration: Ji Yeong Noh, Hye Kwon Kim; Resources: Ji Yeong Noh, Hye Kwon Kim; Supervision: Hye Kwon Kim; Visualization: Ji Yeong Noh; Writing-original draft: Ji Yeong Noh, Hye Kwon Kim.
Figures and Tables
Fig. 1
High-passaged and low-passaged Shaan virus passage scheme in A549, HEK293, and HRT18 cell lines. The original Shaan virus isolate was serially passaged in the MARC145 cell line. Both high-passaged and low-passaged Shaan virus cultures were passaged at an MOI of 0.5 in triplicate across each cell line. A sample from passage 1 of both the high-passaged and low-passaged Shaan virus was subjected to high-throughput sequencing analysis.
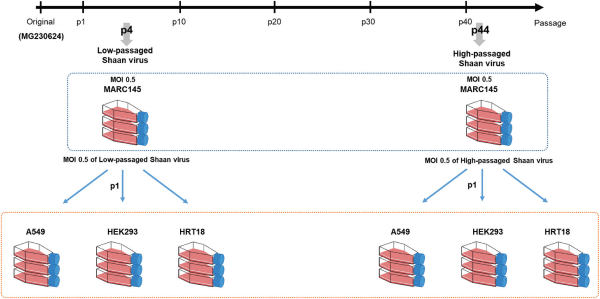
Fig. 2
Viral quantitative analysis from high-passaged and low-passaged Shaan virus passaged in A549, HEK293, HRT18, and MARC145 cell lines. (A) Viral RNAs extracted from the first passage of Shaan virus in each cell line were quantified using real-time RT-PCR targeting the SH region of the virus. (B) The viral antigenome was quantified from the total RNA of each infected cell using reverse transcription and PCR with specifically designed primers. The relative viral RNA and antigenome levels were normalized using the amount of monkey and human GAPDH genes as controls. (C) Viral titers were measured by calculating infectious virus levels (TCID50/ml) in Shaan virus-infected cell lysates. Data were analyzed using one-way ANOVA with Tukey’s multiple comparison test. *p < 0.05; **p < 0.01; ***p < 0.001; ****p < 0.0001; ns, not significant.
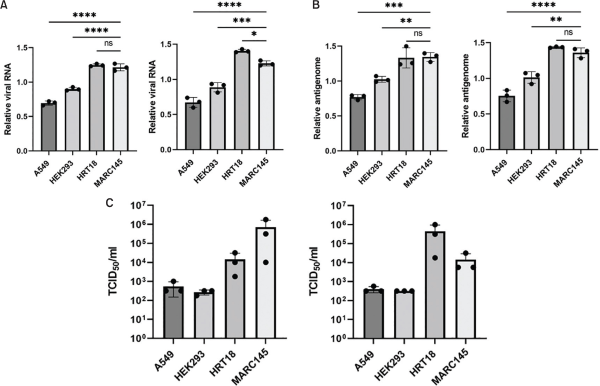
Fig. 3
Differential expression of viral transcript in MARC145, A549, HEK293, and HRT18 cells infected with Shaan virus. The expression of viral gene transcripts, based on transcripts per million mapped reads (TPM), was compared in each cell line infected with 0.5 MOI of high-passaged and low-passaged Shaan virus (passage 1). N, nucleocapsid; P, phosphoprotein; M, matrix protein; F, fusion protein; SH, small hydrophobic protein; TM, transmembrane protein; HN, attachment haemagglutinin neuraminidase glycoprotein; L, large protein.
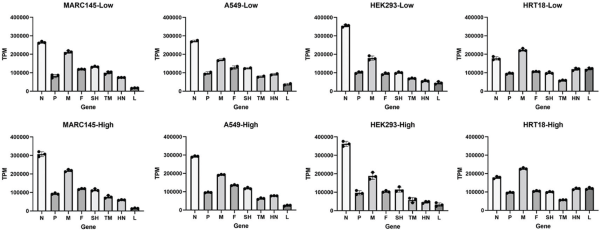
Fig. 4
Comparison of consensus genomic sequence of original and high-passaged and low-passaged Shaan virus in MARC 145 cell. Multiple sequence alignment of consensus genomic sequences was carried out using MAFFT v7.450 integrated into Geneious Prime 2021.2.2. Selected mutations were identified in the F, SH, TM, HN, and L genes. Nucleotide and amino acid numbers (#NT, #AA) were annotated from a reference strain of bat paramyxovirus (GenBank accession no. MG230624).
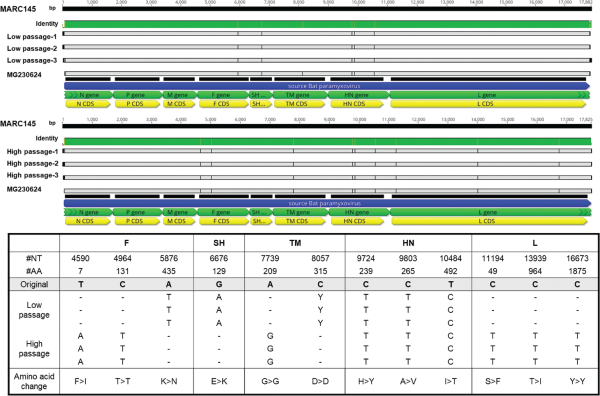
Fig. 5
SNVs found in high-passaged and low-passaged virus. The consensus genomic sequences of the high-passaged (B) and low-passaged (A) Shaan virus, inoculated in MARC145 cells, served as references for SNV analysis. SNVs were analyzed with a minimum variant frequency of 0.25 and 0.05 using Geneious Prime 2021.2.2. SNVs were marked with a red box and annotated with amino acid numbers at each viral gene site. The variant frequency at each SNV is expressed as a percentage value. The final row presents the synonymous or non-synonymous amino acid substitutions detected.
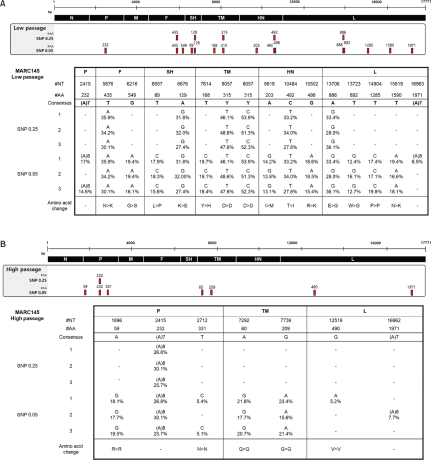
Fig. 6
Comparison of selected mutations found after one passage and in high-passaged and low-passaged Shaan virus-infected cells in human cell line. Nucleotide sequences of high-passaged and low-passaged Shaan virus genes were compared across A549, HEK293, and HRT18 cells that were passaged once. In A549 and HRT18 cells, the selected mutations are highlighted in yellow with the corresponding amino acid number at the mutation site in viral genes. In HEK293 cells, no mutations were observed in the low-passaged Shaan virus. Results from the high-passaged virus in HEK293 cells (grey box) were excluded due to the low read depth (<100×) in mutation analysis. The resulting synonymous or non-synonymous amino acid substitutions are presented in the final row.
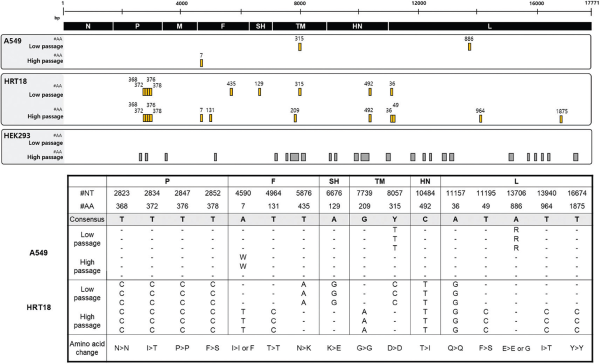
Fig. 7
SNVs found in high-passaged and low-passaged virus after one passage in human cells. The consensus genomic sequence of the high-passaged and low-passaged Shaan virus inoculated in MARC145 cells served as a reference for SNV analysis. SNVs were analyzed at minimum variant frequencies of 0.25 and 0.05 in A549 (A), HEK293 (B), and HRT18 (C) cells. The SNVs are highlighted in red with the corresponding amino acid number at the viral gene site. The variant frequency at each SNV is expressed as a percentage. The SNVs found in the original low-passaged and high-passaged Shaan virus are indicated by blue boxes. In the HEK293 cells, results from the high-passaged Shaan virus-infected group (grey box) were excluded from analysis due to low SNV coverage (<100× read depth). The resulting synonymous or non-synonymous amino acid substitutions are presented in the final row.
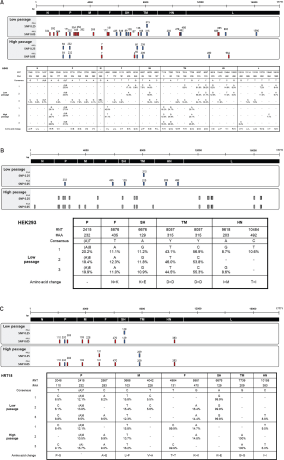
Supplementary Table 1
Sequencing data
Cell name | No. passage | Total reads | Trimmed reads | Mapped reads | Mean read depth (×) | The percentage of reads of phred score (>Q30)* | ||||||||
---|---|---|---|---|---|---|---|---|---|---|---|---|---|---|
|
||||||||||||||
Trimmed reads | Mapped reads | |||||||||||||
MARC145 | p4 (Low passage) |
76155466 | 69476952 | 1630576 | 8280.2 | 98.3% | 98.2% | |||||||
75634052 | 69000772 | 1256180 | 6322.2 | 98.3% | 98.2% | |||||||||
76298278 | 69709920 | 748856 | 3766.0 | 98.3% | 98.2% | |||||||||
p44 (High passage) |
75536994 | 68748356 | 399789 | 2014.8 | 98.3% | 98.2% | ||||||||
76099468 | 69025618 | 411691 | 2069.4 | 98.3% | 98.2% | |||||||||
75747270 | 68793346 | 462013 | 2336.4 | 98.3% | 98.2% | |||||||||
A549 | p1 of low passage |
70276644 | 65154680 | 205120 | 1049.1 | 98.4% | 98.4% | |||||||
57073026 | 48082936 | 125545 | 624.9 | 98.4% | 98.0% | |||||||||
53316092 | 49406698 | 100752 | 517.0 | 98.4% | 98.4% | |||||||||
p1 of high passage |
50285654 | 46592890 | 59306 | 305.0 | 98.4% | 98.4% | ||||||||
54227194 | 49983278 | 93271 | 477.5 | 98.1% | 98.4% | |||||||||
52714662 | 48615816 | 156932 | 802.1 | 98.4% | 98.3% | |||||||||
HEK293 | p1 of low passage |
75796120 | 71982628 | 1031234 | 5434.7 | 98.7% | 98.7% | |||||||
75707030 | 71871186 | 867148 | 4553.6 | 98.7% | 98.6% | |||||||||
73091564 | 69472950 | 1221750 | 6452.6 | 98.7% | 98.7% | |||||||||
p1 of high passage |
74771938 | 70963538 | 1800 | 9.4 | 98.7% | 98.7% | ||||||||
76279780 | 72405440 | 3256 | 17.0 | 98.7% | 98.7% | |||||||||
76111894 | 71953910 | 2976 | 15.6 | 98.7% | 98.7% | |||||||||
HRT18 | p1 of low passage |
58328530 | 53270462 | 465262 | 2387.8 | 98.5% | 98.4% | |||||||
59752932 | 54840618 | 397711 | 2059.2 | 98.6% | 98.5% | |||||||||
53310344 | 48593456 | 392797 | 2014.9 | 98.5% | 98.4% | |||||||||
p1 of high passage |
55034724 | 49700814 | 470425 | 2384.0 | 98.3% | 98.2% | ||||||||
61325484 | 55897850 | 431535 | 2217.6 | 98.5% | 98.4% | |||||||||
59167894 | 53474980 | 430264 | 2177.2 | 98.4% | 98.2% | |||||||||
Mean |
Supplementary Table 2
The raw data in SNV analysis in low- and high-passaged Shaan viruses after one passage in A549 cells
Gene | Position | Nucleotide change |
Amino acid change | Coverage | Variant frequency (%) | |||||||||||
---|---|---|---|---|---|---|---|---|---|---|---|---|---|---|---|---|
|
||||||||||||||||
#NT | #AA | |||||||||||||||
|
||||||||||||||||
A549-low passaged virus | ||||||||||||||||
1 | P | 2415 | 232 | (A)7→(A)8 | - | - | 1006 | 23.90% | ||||||||
F | 5876 | 435 | T→A | AAT>AAA | N>K | 1305 | 17.10% | |||||||||
6216 | 549 | G→A | GGC>AGC | G>S | 1234 | 5.90% | ||||||||||
SH | 6557 | 89 | T→C | CTA>CCA | L>P | 1278 | 7.50% | |||||||||
6676 | 129 | A→G | AAA>GAA | K>E | 1454 | 14.60% | ||||||||||
TM | 7614 | 168 | T→C | TAT>CAT | Y>H | 797 | 8.00% | |||||||||
8057 | 315 | Y→T | GAY>GAT | D>D | 976 | 61.80% | ||||||||||
Y→C | GAY>GAC | D>D | 976 | 38.20% | ||||||||||||
HN | 9618 | 203 | A→G | ATA>ATG | I>M | 1073 | 9.20% | |||||||||
10484 | 492 | C→T | ACT>ATT | T>I | 1143 | 14.90% | ||||||||||
10502 | 498 | G→A | AGA>AAA | R>K | 1198 | 8.30% | ||||||||||
L | 13723 | 892 | T→G | TGG>GGG | W>G | 2674 | 7.20% | |||||||||
15819 | 1590 | T→A | AAT>AAA | N>K | 4770 | 8.40% | ||||||||||
2 | N | 1045 | 334 | G→C | GCT>CCT | A>P | 176 | 7.40% | ||||||||
1219 | 392 | T→C | TTG>CTG | L>L | 302 | 5.60% | ||||||||||
1437 | 464 | (A)6→(A)7 | - | - | 298 | 6.70% | ||||||||||
P | 2248 | 2316 | G→A | GTT>ATT | V>I | 237 | 5.90% | |||||||||
2415 | 232 | (A)7→(A)8 | - | - | 66 | 16.70% | ||||||||||
2706 | 329 | A→G | GAA>GAG | E>E | 95 | 11.60% | ||||||||||
2719 | 334 | A→G | AAG>GAG | K>E | 105 | 11.40% | ||||||||||
2754 | 345 | T→C | TCT>TCC | S>S | 127 | 7.90% | ||||||||||
2793 | 358 | A→G | ATA>ATG | I>M | 151 | 8.60% | ||||||||||
2863 | 382 | A→G | ATT>GTT | I>V | 149 | 8.10% | ||||||||||
M | 4152 | 265 | G→DEL | - | - | 264 | 6.10% | |||||||||
4398 | 347 | A→G | AAG>GAG | K>E | 68 | 5.90% | ||||||||||
F | 5085 | 172 | T→C | TTA>CTA | L>L | 166 | 5.40% | |||||||||
5113 | 181 | T→C | ATA>ACA | I>T | 145 | 6.20% | ||||||||||
SH | 6676 | 129 | A→G | AAA>GAA | K>E | 8220 | 15.40% | |||||||||
6851 | 187 | C→T | CCA>CTA | P>L | 184 | 6.00% | ||||||||||
TM | 7518 | 136 | T→C | TGG>CGG | W>R | 110 | 8.20% | |||||||||
7614 | 168 | T→C | TAT>CAT | Y>H | 164 | 10.40% | ||||||||||
8057 | 315 | Y→T | GAY>GAT | D>D | 119 | 64.70% | ||||||||||
Y→C | GAY>GAC | D>D | 119 | 35.30% | ||||||||||||
8682 | 524 | A→G | AAA>GAA | K>E | 45 | 22.20% | ||||||||||
HN | 9252 | 82 | AC→DEL | - | - | 77 | 36.40% | |||||||||
10442 | 478 | T→C | CTA>CCA | L>P | 110 | 11.80% | ||||||||||
10484 | 492 | C→T | ACT>ATT | T>I | 98 | 9.20% | ||||||||||
L | 12316 | 423 | (A)5→(A)4 | - | - | 36 | 22.20% | |||||||||
13114 | 689 | T→C | TTG>CTG | L>L | 163 | 7.40% | ||||||||||
13133 | 695 | T→C | TTA>TCA | L>S | 126 | 7.90% | ||||||||||
13609 | 854 | A→C | AAA>CAA | K>Q | 36 | 33.30% | ||||||||||
13723 | 892 | T→G | TGG>GGG | W>G | 73 | 20.50% | ||||||||||
16158 | 1703 | A→G | ATA>ATG | I>M | 89 | 5.60% | ||||||||||
16175 | 1709 | A→G | AAG>AGG | K>R | 77 | 6.50% | ||||||||||
16178 | 1710 | A→G | AAA>AGA | K>R | 79 | 6.30% | ||||||||||
16189 | 1714 | A→G | ATG>GTG | M>V | 72 | 6.90% | ||||||||||
16229 | 1727 | A→G | TAT>TGT | Y>C | 78 | 11.50% | ||||||||||
16272 | 1741 | A→G | GAA>GAG | E>E | 57 | 8.80% | ||||||||||
3 | P | 2415 | 232 | (A)7→(A)8 | - | - | 448 | 20.80% | ||||||||
F | 5876 | 435 | T→A | AAT>AAA | N>K | 1305 | 14.80% | |||||||||
SH | 6676 | 129 | A→G | AAA>GAA | K>E | 675 | 15.60% | |||||||||
TM | 8057 | 315 | Y→T | GAY>GAT | D>D | 460 | 68.30% | |||||||||
Y→C | GAY>GAC | D>D | 460 | 31.70% | ||||||||||||
HN | 9618 | 203 | A→G | ATA>ATG | I>M | 610 | 12.60% | |||||||||
10484 | 492 | C→T | ACT>ATT | T>I | 618 | 17.20% | ||||||||||
10502 | 498 | G→A | AGA>AAA | R>K | 677 | 5.30% | ||||||||||
L | 13723 | 892 | T→G | TGG>GGG | W>G | 191 | 11.00% | |||||||||
15819 | 1590 | T→A | AAT>AAA | N>K | 194 | 6.70% | ||||||||||
A549-high passaged virus | ||||||||||||||||
1 | P | 1896 | 59 | A→G | AGA>AGG | R>R | 399 | 29.10% | ||||||||
2415 | 232 | (A)7→(A)8 | - | - | 273 | 27.50% | ||||||||||
F | 4590 | 7 | A→T | I>L | 285 | 42.80% | ||||||||||
TM | 7119 | 3 | T→C | TAT>CAT | Y>H | 143 | 18.20% | |||||||||
7292 | 60 | A→G | CAA>CAG | Q>Q | 154 | 24.70% | ||||||||||
7739 | 209 | G→A | GGG>GGA | G>G | 178 | 28.70% | ||||||||||
L | 16666 | 1873 | T→C | TTT>CTT | F>L | 92 | 5.40% | |||||||||
16674 | 1875 | T→C | TAT>TAC | Y>Y | 96 | 8.30% | ||||||||||
2 | P | 1896 | 59 | A→G | AGA>AGG | R>R | 673 | 26.70% | ||||||||
2415 | 232 | (A)7→(A)8 | - | - | 469 | 25.40% | ||||||||||
F | 4590 | 7 | A→T | ATA>TTA | I>L | 521 | 41.10% | |||||||||
TM | 7119 | 3 | T→C | TAT>CAT | Y>H | 188 | 13.80% | |||||||||
7292 | 60 | A→G | CAA>CAG | Q>Q | 321 | 34.90% | ||||||||||
7739 | 209 | G→A | GGG>GGA | G>G | 295 | 28.10% | ||||||||||
L | 13940 | 964 | T→C | ATA>ACA | I>T | 112 | 7.10% | |||||||||
3 | P | 1896 | 59 | A→G | AGA>AGG | R>R | 1107 | 26.60% | ||||||||
2415 | 232 | (A)7→(A)8 | - | - | 776 | 31.30% | ||||||||||
TM | 7119 | 3 | T→C | TAT>CAT | Y>H | 407 | 15.00% | |||||||||
7292 | 60 | A→G | CAA>CAG | Q>Q | 589 | 28.90% | ||||||||||
7739 | 209 | G→A | GGG>GGA | G>G | 509 | 26.50% | ||||||||||
L | 12519 | 490 | G→A | GTG>GTA | V>V | 267 | 6.40% |
Supplementary Table 3
The raw data in SNV analysis in low- and high-passaged Shaan viruses after one passage in HEK293 cells
Gene | Position | Nucleotide change |
Amino acid change | Coverage | Variant frequency (%) | |||||||||||
---|---|---|---|---|---|---|---|---|---|---|---|---|---|---|---|---|
|
||||||||||||||||
#NT | #AA | |||||||||||||||
|
||||||||||||||||
HEK293-low passaged virus | ||||||||||||||||
1 | P | 2415 | 232 | (A)7→(A)8 | - | - | 5881 | 20.20% | ||||||||
F | 5876 | 435 | T→A | AAT>AAA | N>K | 4434 | 11.10% | |||||||||
SH | 6676 | 129 | A→G | AAA>GAA | K>E | 6329 | 11.20% | |||||||||
TM | 8057 | 315 | Y→T | GAY>GAT | D>D | 4128 | 43.10% | |||||||||
Y→C | GAY>GAC | D>D | 4128 | 56.90% | ||||||||||||
HN | 9618 | 203 | A→G | ATA>ATG | I>M | 3385 | 8.70% | |||||||||
10484 | 492 | C→T | ACT>ATT | T>I | 3470 | 10.60% | ||||||||||
2 | P | 2415 | 232 | (A)7→(A)8 | - | - | 3895 | 18.40% | ||||||||
F | 5876 | 435 | T→A | AAT>AAA | N>K | 3662 | 12.30% | |||||||||
SH | 6676 | 129 | A→G | AAA>GAA | K>E | 4731 | 11.80% | |||||||||
TM | 8057 | 315 | Y→T | GAY>GAT | D>D | 2871 | 46.00% | |||||||||
Y→C | GAY>GAC | D>D | 2871 | 53.80% | ||||||||||||
3 | P | 2415 | 232 | (A)7→(A)8 | - | - | 6435 | 19.90% | ||||||||
F | 5876 | 435 | T→A | AAT>AAA | N>K | 5244 | 11.00% | |||||||||
SH | 6676 | 129 | A→G | AAA>GAA | K>E | 7214 | 10.90% | |||||||||
TM | 8057 | 315 | Y→T | GAY>GAT | D>D | 4349 | 44.50% | |||||||||
Y→C | GAY>GAC | D>D | 4349 | 55.30% | ||||||||||||
HN | 9618 | 203 | A→G | ATA>ATG | I>M | 3793 | 8.60% | |||||||||
HEK293-high passaged virus | ||||||||||||||||
1 | N | 279 | 78 | T→C | TCT>TCC | S>S | 47 | 6.40% | ||||||||
P | 1908 | 63 | T→C | CAT>CAC | H>H | 14 | 21.40% | |||||||||
2342 | 208 | C→T | CCC>CTC | P>L | 6 | 33.30% | ||||||||||
2415 | 232 | (A)7→(A)8 | - | - | 5 | 80.00% | ||||||||||
F | 4590 | 7 | A→T | ATA>TTA | I>L | 12 | 33.30% | |||||||||
HN | 12113 | 355 | (T)7→(T)8 | - | - | 6 | 33.30% | |||||||||
2 | P | 1896 | 59 | A→G | AGA>AGG | R>R | 22 | 36.40% | ||||||||
2415 | 232 | (A)7→(A)8 | - | - | 22 | 27.30% | ||||||||||
M | 3851 | 164 | (T)3→(T)4 | - | - | 45 | 8.90% | |||||||||
F | 4590 | 7 | A→T | ATA>TTA | I>L | 13 | 69.20% | |||||||||
SH | 6563 | 91 | (A)6→(A)7 | - | - | 22 | 45.50% | |||||||||
3 | P | 1896 | 59 | A→G | AGA>AGG | R>R | 20 | 25.00% | ||||||||
2415 | 232 | (A)7→(A)8 | - | - | 13 | 23.10% | ||||||||||
2712 | 331 | T→C | AAT>AAC | N>N | 13 | 30.80% | ||||||||||
M | 3491 | 44 | C→T | ATA>TTA | I>L | 28 | 10.70% | |||||||||
TM | 7292 | 60 | A→G | CAA>CAG | Q>Q | 15 | 40.00% | |||||||||
7394 | 94 | G→A | CCG>CCA | P>P | 9 | 44.40% | ||||||||||
8751 | 547 | T→C | TAC>CAC | Y>H | 37 | 10.80% | ||||||||||
L | 12684 | 545 | A→G | AAA>AAG | K>K | 5 | 80.00% | |||||||||
12691 | 548 | A→G | AGA>GGA | R>G | 7 | 57.10% | ||||||||||
12919-12920 | 624 | AA→GG | AAC>GGC | N>G | 8 | 50.00% | ||||||||||
12928 | 627 | A→G | ACA>GCA | T>A | 9 | 44.40% | ||||||||||
12955-12956 | 636 | AA→GG | AAA>GGA | K>G | 9 | 44.40% | ||||||||||
12964 | 639 | A→G | AGA>GGA | R>G | 9 | 44.40% | ||||||||||
16088 | 1680 | G→A | AGA>AAA | R>K | 10 | 60.00% | ||||||||||
16490 | 1814 | A→G | TAC>TGC | Y>C | 4 | 50.00% | ||||||||||
16500 | 1817 | A→G | TCA>TCG | S>S | 5 | 40.00% |
Supplementary Table 4
The raw data in SNV analysis in low- and high-passaged Shaan viruses after one passage in HRT18 cells
Gene | Position | Nucleotide change | Amino acid change | Coverage | Variant frequency (%) | |||||||||||
---|---|---|---|---|---|---|---|---|---|---|---|---|---|---|---|---|
|
||||||||||||||||
#NT | #AA | |||||||||||||||
|
||||||||||||||||
HRT18-low passaged virus | ||||||||||||||||
1 | P | 2048 | 110 | T→C | TTT>TCT | F>S | 1424 | 6.50% | ||||||||
2415 | 232 | (A)7→(A)8 | - | - | 1410 | 12.10% | ||||||||||
2567 | 283 | C→A | GCG>GAG | A>E | 1765 | 8.20% | ||||||||||
M | 3666 | 103 | C→T | CTT>TTT | L>F | 4935 | 15.80% | |||||||||
4042 | 228 | T→C | GTC>GCC | V>A | 3945 | 5.50% | ||||||||||
SH | 6676 | 129 | A→G | AAA>GAA | K>E | 2029 | 99.90% | |||||||||
2 | P | 2048 | 110 | T→C | TTT>TCT | F>S | 1449 | 6.10% | ||||||||
2415 | 232 | (A)7→(A)8 | - | - | 1281 | 13.80% | ||||||||||
M | 3666 | 103 | C→T | CTT>TTT | L>F | 4856 | 15.40% | |||||||||
4042 | 228 | T→C | GTC>GCC | V>A | 3715 | 5.60% | ||||||||||
F | 5981 | 470 | G→A | AAG>AAA | K>K | 1935 | 16.40% | |||||||||
SH | 6676 | 129 | A→G | AAA>GAA | K>E | 1594 | 99.90% | |||||||||
3 | P | 2048 | 110 | T→C | TTT>TCT | F>S | 1270 | 8.80% | ||||||||
2415 | 232 | (A)7→(A)8 | - | - | 1229 | 9.50% | ||||||||||
2567 | 283 | C→A | GCG>GAG | A>E | 1673 | 9.50% | ||||||||||
M | 3666 | 103 | C→T | CTT>TTT | L>F | 3984 | 12.30% | |||||||||
F | 5981 | 470 | G→A | AAG>AAA | K>K | 1444 | 99.80% | |||||||||
HN | 10158 | 383 | C→T | ATC>ATT | I>I | 1746 | 8.80% | |||||||||
HRT18-high passaged virus | ||||||||||||||||
1 | P | 2048 | 110 | T→C | TTT>TCT | F>S | 1554 | 8.40% | ||||||||
2415 | 232 | (A)7→(A)8 | - | - | 1412 | 12.10% | ||||||||||
M | 3666 | 103 | C→T | CTT>TTT | L>F | 5144 | 15.80% | |||||||||
F | 4964 | 131 | T→C | ACT>ACC | T>T | 1875 | 99.90% | |||||||||
5981 | 470 | G→A | AAG>AAA | K>K | 2184 | 14.70% | ||||||||||
HN | 10158 | 383 | C→T | ATC>ATT | I>I | 1866 | 9.50% | |||||||||
2 | P | 2415 | 232 | (A)7→(A)8 | - | - | 1322 | 10.50% | ||||||||
2567 | 283 | C→A | GCG>GAG | A>E | 1668 | 9.90% | ||||||||||
M | 3666 | 103 | C→T | CTT>TTT | L>F | 4768 | 13.70% | |||||||||
F | 5981 | 470 | G→A | AAG>AAA | K>K | 1770 | 14.80% | |||||||||
TM | 7739 | 209 | G→A | GGG>GGA | G>G | 410 | 100% | |||||||||
3 | P | 2048 | 110 | T→C | TTT>TCT | F>S | 1339 | 9.10% | ||||||||
2415 | 232 | (A)7→(A)8 | - | - | 1366 | 15.70% | ||||||||||
2567 | 283 | C→A | GCG>GAG | A>E | 1745 | 9.00% | ||||||||||
3666 | 103 | C→T | CTT>TTT | L>F | 5053 | 16.20% | ||||||||||
F | 4964 | 131 | T→C | ACT>ACC | T>T | 1792 | 99.90% | |||||||||
TM | 7739 | 209 | G→A | GGG>GGA | G>G | 453 | 100% | |||||||||
HN | 10158 | 383 | C→T | ATC>ATT | I>I | 1531 | 6.00% |
Supplementary Table 5
Primer and probe of target gene detection with real time RT-PCR
Target gene | Name | Seq. (5′-3′) | Ref. | |||
---|---|---|---|---|---|---|
For detection of viral RNA | ||||||
Shaan virus | PVM-F | CCCAGGAGTATGGTTATCAAGTGAGG | ||||
SH gene | PVM-R | TCCATTGGGCTCTCTTTGTTTGC | ||||
PVM-P | FAM-CCCATCCCAGACCAGCCACCAGACCC-TAMRA | |||||
For detection of antigenome | ||||||
primer for cDNA synthesis | N-P cD primer | GAGCATCCAGAGACTTCCAGTGACGTTTGGCTTTGTCTG | ||||
Shaan virus antigenom | BPV-NP-F | GGATTAACCTCAAAACCAATGC | ||||
BPV-tail-R | GAGCATCCAGAGACTTCCA | |||||
BPV-NP-P | FAM-AAAGTGACATAGGCCAAGCTC-BHQ1 | |||||
Human GAPDH gene | hGAPDH-F | GAAGGTGAAGGTCGGAGTC | Das et al., 2000 | |||
hGAPDH-R | GAAGATGGTGATGGGATTTC | |||||
hGAPDH-P | HEX-CAAGCTTCCCGTTCTCAGCC-BHQ1 | |||||
Monkey GAPDH gene | mGAPDH-F | TGACAACAGCCTCAAGATCG | Shi et al., 2016 | |||
mGAPDH-R | GTCTTCTGGGTGGCAGTGAT | |||||
mGAPDH-P | HEX-TGGAAGGACTCATGACCACA-BHQ1 |