Introduction
Modern Biotechnology now plays a crucial role in addressing various global issues such as food security, virus outbreaks, and climate change (Adugnaw & Tileye, 2024). Living modified organisms (LMOs), which comprise organisms containing newly recombined genetic material, are developed through biotechnology. Living modified (LM) crops, which emerged in the 1990s to address food security and climate change issues, were developed by inserting genetic material with specific enhanced functions into plants (James & Anatole, 1996). According to the ISAAA report, between 2000 and 2019, the global cultivation area of major LM crops such as soybeans, maize, cotton, and canola increased steadily by 3.5-fold, 5.9-fold, 4.8-fold, and 3.6-fold, respectively. This increase in cultivation area reflects both the rising demand for LM crops and the growing potential risks associated with LMOs. A review of domestic LMO import approvals reveals that, since 2018, over 10 million tons of LM crops have been imported annually for food and feed purposes in South Korea (KBCH, 2023).
Among these, LMO maize (Zea mays L.) constitutes a significant portion of global maize production, with its cultivation expanding annually, particularly in major producing countries such as the United States, Brazil, and Argentina (ISAAA, 2019).
In South Korea, the import and use of LM maize have steadily increased, driven by the growing demand for maize in the food and feed industries (KBCH, 2021). However, the widespread adoption of LM maize has raised concerns regarding the unintentional release of these organisms into the environment, which could pose risks to the natural ecosystem. As a result, there is a critical need for effective methods to detect and monitor LM maize to ensure its safe management and prevent unintended environmental impacts.
To address this requirement, we developed a multiplex PCR method that is capable of simultaneously detecting four LM maize events (DP-004114-3, MON87403, MON88017, and MON89034), all of which have received approval for use in South Korea. The DP-004114-3 event in LM maize was engineered to convey various resistances (glufosinate herbicide tolerance, Coleopteran insect resistance, and Lepidopteran insect resistance) by incorporating the Bacillus thuringiensis genes (cry1F, cry34Ab1, cry35Ab1, and pat). The MON87403 event produces the AtHB17 protein from Arabidopsis thaliana, enhancing ear biomass. The MON88017 event, developed by Monsanto, produces the CP4-EPSPS and Cry3Bb1 proteins from Agrobacterium tumefaciens and B. thuringiensis, respectively, offering resistance to glyphosate herbicides and Coleopteran insects. The MON89034 event incorporates cry2Ab2 and cry1A.105 genes from B. thuringiensis to confer resistance against Lepidopteran insects. We obtained the genetic sequences specific to these events from authoritative sources, such as the European Commission-Joint Research Centre and the Animal and Plant Quarantine Agency. By designing event-specific primers, we established a reliable multiplex PCR method, validated through specificity assays, random certified reference material (CRM) mixture analyses, and limit of detection (LOD) tests.
The developed multiplex PCR method was further tested on suspicious samples gathered during the LMO monitoring and post-management project. The results demonstrated the method’s effectiveness in detecting specific LMO maize events, confirming its potential application in routine LMO monitoring, quarantine inspections, and food safety analyses. This method not only facilitates the efficient identification of LMO maize but also contributes to broader efforts to safeguard South Korea’s natural ecosystem from unintended LMO releases.
Materials and Methods
Plant materials and DNA extraction
The CRMs used as controls in the PCR reactions were procured from the American Oil Chemist’s Society (AOCS; Urbana, IL, USA) and the Institute for Reference Materials and Measurements (IRMM; Geel, Belgium, Table 1). Plant samples originated from the LMO environmental monitoring project in 2021 and 2022. Genomic DNAs (gDNAs) from powdered target event CRMs and LM maize leaves were extracted using the Libex NP968 (Tianlong, China). This extraction followed the standard protocol of Tianlong for plant gDNA extraction. The resulting genomic DNA was measured with a NanoDrop ND2000 (Thermo Scientific, USA), diluted to a concentration of 50 ng μL–1, and stored at –20°C until needed for PCR analysis.
Primer design for multiplex PCR analysis
The nucleotide sequence for the maize endogenous gene Alcohol Dehydrogenase 1 (Adh1, GenBank accession no. AF123535) was sourced from NCBI GenBank for its use as a positive control in multiplex PCR, and primers were accordingly designed. The design of specific amplification primers for each LM maize gene cassette and its genome employed information from the Joint Research Centre-European Commission (JRC-EC). All primers were synthesized by Biofact Inc. (Daejeon, Republic of Korea), diluted to 5 pmole μL–1, and used in the experiments (Table 2).
Event-specific PCR analysis
The multiplex PCR reaction mixture included 15 μL of 2X Lamp Taq PCR Pre-Mix (Biofact Inc., Daejeon, Republic of Korea), 50 ng of genomic DNA, and each primer at a final concentration of 0.16 μM. The total volume was adjusted to 30 μL with distilled water. The amplification targeted inserted LMO genes in CRMs and suspicious plants using the ProFlex PCR System (Applied Biosystems, Waltham, MA, USA) under conditions: (1) initial denaturation at 95°C for 5 min, (2) denaturation (33 cycles) at 95°C for 30 s, (3) annealing at 63°C for 30 s, (4) extension at 72°C for 30 s, (5) final extension at 72°C for 7 min, and (6) hold at 4°C. The PCR products were analyzed by electrophoresis on a 2.5% agarose gel and visualized using the ChemiDoc XRS+ Imaging System (Bio-Rad, Hercules, CA, USA). Nucleotide sequencing of the PCR products was conducted by Biofact Inc. (Daejeon, Republic of Korea) to identify the event name, maker, and trait type. Sequences were aligned using BioEdit v7.2.6.0. All PCR experiment involved three independent biological replications.
Specificity and sensitivity of multiplex PCR assay
To ascertain the specificity of the developed multiplex PCR technique, we conducted experiments using three non-LM and eleven LM maize CRMs, including four event CRMs. Additionally, we created combinations of single (four types) and hypothetical hybrid progenies (eleven types) using four LM maize CRMs and performed multiplex PCR amplification experiments. The final template DNA amount for the PCR reactions was set at 200 ng, supplemented with genomic DNA from non-LMO as needed. In the limit of detection (LOD) analysis for the newly developed multiplex PCR, we prepared samples mixed with four CRM DNAs at an initial concentration of 100 ng μL–1 and sequentially diluted them 1:2 using sterilized distilled water. Each dilution served as a template in the multiplex PCR.
Application for LMO identification in collected suspicious maize samples
DNA was extracted from suspicious maize samples collected during LMO monitoring in 2021 and 2022 to identify unintentionally released LM maize plants. The PCR conditions were consistent with those described above. PCR products were subjected to electrophoresis using a 2.5% agarose gel at 135V for 20 minutes, and images were captured using the Chemi-DocTM XRS+ (Bio-Rad, USA). Each band amplified by the multiplex PCR was sent to Biofact Inc. (Daejeon, Republic of Korea) for nucleotide sequencing to confirm the amplification results (data not shown).
Results and Discussion
Primer design for multiplex PCR analysis
A critical aspect of developing PCR-based methods for detecting LMOs qualitatively and quantitatively involves sourcing CRMs from which template DNA is extracted. These CRMs are developed and distributed by organizations such as the Institute for Reference Materials and Measurements (IRMM) in Europe and the American Oil Chemists’ Society (AOCS) in the United States. We sourced all necessary CRMs for non-LM and LM maize from IRMM and AOCS to ascertain the specificity of the multiplex PCR we developed.
The multiplex PCR technique for identifying LMOs is an effective approach that reduces both time and cost when analyzing multiple samples. The NIE has developed this method for major approved LM crops, including maize, cotton, soybeans, canola, and alfalfa (Jo et al., 2016; Shin et al., 2016; Eum et al., 2019; Kim et al., 2019; Choi et al., 2020; Kim et al., 2020; Park et al., 2020; Kim et al., 2021). In this study, we investigated the genetic information of four LM maize events to identify the inserted genes and nearby genome sequences. Using this data, we developed several sets of event-specific primers (Fig. 1). Each primer specifically amplified its target sequence in simplex PCR assays, thus laying the foundation for developing a multiplex PCR for LM maize (Fig. 2).
When designing primers for both simplex and multiplex PCR, it is crucial to prevent primer dimer formation to avoid non-specific PCR product amplification. Factors such as high primer concentrations, cold-start PCR, and low-efficiency PCR enzymes can contribute to primer dimer formation (Brownie et al., 1997). To mitigate this issue, we evaluated various primer combinations using the Multiple Primer Analyzer (ThermoFisher, MA, USA). We then selected primer sets that showed differences in amplification product sizes, subsequently using them in multiplex PCR experiments.
Establishment of LM maize multiplex PCR method and verification of specificity
Using the optimally selected primer set, we conducted multiplex PCR experiments on four LM maize events. The outcomes, observed through 2.5% agarose gel electrophoresis, demonstrated specific amplification for the DP-004114-3 (218 bp), MON89034 (316 bp), MON88017 (410 bp), and MON87403 (464 bp) events (Fig. 2). Each PCR product was sequenced, verifying the sequences of the respective events (data not shown). The developed multiplex PCR produced bands ranging from 218 to 464 bp, with size disparities of about 50 to 100 bp between the bands of each event, enabling clear differentiation on a 2.5% agarose gel.
Typically, primer concentrations in multiplex PCR experiments vary from 0.1 to 0.5 μM, adjusted according to the DNA copy number and structural complexity (Markoulatos et al., 2002). However, adjusting primer concentrations for each event improves multiplex PCR efficiency but adds complexity to the experimental process, potentially limiting its practical application.
To overcome the limitations of previous techniques, we developed a multiplex PCR assay utilizing primers at a uniform concentration of 0.16 μM. This approach simplified the experimental procedure while ensuring efficient amplification across all targets.
The developed multiplex PCR will be employed to qualitatively analyze LMO-suspicious samples collected from NIE’s LMO environmental monitoring project. To verify the specificity of the newly developed LM maize multiplex PCR, we conducted specificity validation experiments using CRMs. In the multiplex PCR using genomic DNA from three non-LM maize samples and eleven LM maize events, only the internal PCR control gene (Adh1) was amplified, and no other bands were observed in all CRMs (Fig. 2). This confirms that the newly developed LM maize multiplex PCR method is specific to the four LM maize events.
Efficiency of the newly developed multiplex PCR method
To validate the efficacy of the newly developed multiplex detection method for detecting four LM maize events, we tested whether various hypothetical progeny combinations would be specifically amplified by combining four samples (randomly mixed). The analysis confirmed the presence of specific event amplification bands in six mixtures of two CRM samples and four mixtures of three CRM samples (Fig. 3).
Recent trends indicate that most imported LMO events are of stack types, and as their environmental distribution expands, the unintended release of LMOs into the domestic ecosystem is also increasing (NIE, 2023). The multiplex PCR method developed in this study will be utilized to analyze LM maize events that have been unintentionally released into the natural environment. The successful validation of this method using hypothetical combinations enhances its potential application for detecting LMOs with mixed gene cassettes in future environmental monitoring efforts. We developed a multiplex PCR method for four LM maize events, and its efficiency was confirmed by testing random mixed CRM samples. These results showed that all future stacks might be detected by this multiplex PCR method, and that the detection efficiency is quite reliable for analyzing LM maize monitoring samples.
Limit of detection (LOD) verification
The multiplex PCR for LM maize, designed for analyzing suspicious samples in the LMO monitoring project and applications such as quarantine, must efficiently perform PCR analysis with minimal DNA samples across various types and conditions (Eum et al., 2019). To ascertain the LOD of this LM maize multiplex PCR method, we conducted serial dilutions of the CRMs for four events to concentrations of 100, 50, 25, 12.5, 6.6, 3.1, 1.6, and 0.8 ng μL–1. It was found that detection down to a concentration of 12.5 ng μL–1 was possible on agarose gel electrophoresis (Fig. 4).
PCR-based LMO detection technologies are highly accurate, regardless of sample condition and quantity, and are extensively used in detecting various LM crops. The developed multiplex PCR for the four LM maize events is highly sensitive, detecting each event with minimal DNA samples. This technology is anticipated to be employed in future LMO environmental monitoring, import agricultural product quarantine, and food ingredient analysis.
Application for LMO identification in collected suspicious samples
We further confirmed the efficacy of the developed multiplex PCR in analyzing suspicious samples from the LMO monitoring and post-management project. The analysis of 17 LMO-suspicious samples showed specific amplification bands for each maize sample (Fig. 5). As indicated in Fig. 5, eight samples were identified as LM maize using the new multiplex PCR method, and their event names were disclosed. Nine samples (2021-3, 2021-7, 2021-8, 2021-10, 2021-11, 2022-1, 2022-2, 2022-5, and 2022-6) were identified as a different type of LM maize (data not shown). Specifically, of the four events targeted, only MON88017 and MON89034 were found to have been unintendedly released through transport routes and harbors in 2021 and 2022. The other events, DP-004114-3 and MON87403, had not been released during the monitoring periods in 2021 and 2022. These findings suggest that our novel approach could aid in managing and/or physically eliminating the specific LM maize events identified in these unintentional releases.
Conclusion
In this study, we developed and validated a multiplex PCR method for the simultaneous detection of four specific LM maize events (DP-004114-3, MON89034, MON88017, and MON87403), approved for use in South Korea. The method exhibited high specificity and sensitivity, capable of detecting low concentrations of template DNA and accurately identifying target events in mixed samples. Our findings demonstrate that this multiplex PCR assay is a reliable and efficient tool for the monitoring and management of LMOs, especially in environmental surveillance and regulation.
The application of this method in analyzing suspicious LM samples collected during the LMO monitoring project further underscores its practical utility in identifying unintentional LMO releases. By enabling precise detection of LM maize events, the developed assay provides critical support for protecting South Korea’s natural ecosystems against potential risks associated with LMO dissemination. Furthermore, this method could be adapted and expanded to monitor other LMO crops, contributing to broader efforts in LMO management and biosafety.
Overall, the multiplex PCR technique developed in this study marks a significant advancement in LMO detection methodologies, offering a robust approach to ensuring the safe use and management of LMOs in agriculture and environmental protection.
Author Contributions
WC designed the experiment and wrote the paper, while AMY performed the experiments.
References
KBCH (2021, 23 September 2024) Korea Biosafety Clearing House KBCH Trend Report, Available from: http://biosafety.or.kr/, accessed on
KBCH (2023, 23 September 2024) Korea Biosafety Clearing House KBCH Trend Report, Available from: http://biosafety.or.kr/, accessed on
Figures and Tables
Fig. 1
Schematic diagrams of transgene structures and event-specific primer positions for four LM maize events. Transgene forms and event-specific primer binding positions of DP-004114-3 (A), MON89034 (B), MON88017 (C), and MON87403 (D) are illustrated. The bold lines depict the flanking region of the maize genome. (RB: Right border; LB: Left border; V: vector binding primer, P: maize genome binding primer; gray pentagon: Promoter; Open Square: coding gene; gray square: 3’ terminator). Arrows indicate primer binding locations.
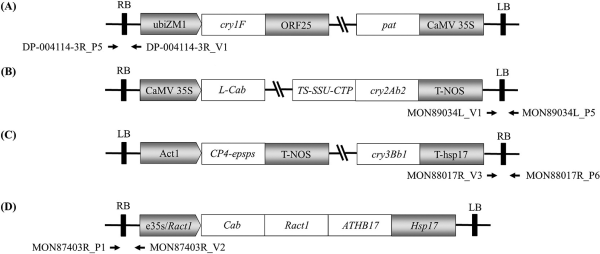
Fig. 2
Optimization of LM maize multiplex PCR assay and verification of specificity. Results of the Multiplex PCR and verification of specificity using maize genomic DNA (lane 1, DP-004114-3; lane 2, MON89034; lane 3, MON88017; lane 4, MON87403; lane 5, mixed 4 maize CRMs; lane 6, non-LM maize (0406-A2); lane 7, non-LM maize (0407-A); lane 8, non-LM maize (0411-C2); lane 9, Bt11; lane 10, DAS-59122-7; lane 11, MON87427; lane 12, MON863; lane 13, TC1507; lane 14, VCO-01981-5; lane 15, DP-098140-6; lane 16, MON810). M represents a 100 bp DNA size marker. The maize endogenous gene (Adh1) was used for PCR control.
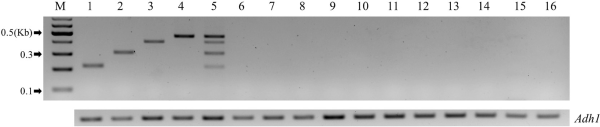
Fig. 3
Efficiency test of maize multiplex PCR using a random CRM DNA mixture. Each lane represents lane 1: non-LM maize DNA (0406-A2); lanes 2–16: random mixtures of LM maize CRMs. M represents a 100 bp DNA size marker.
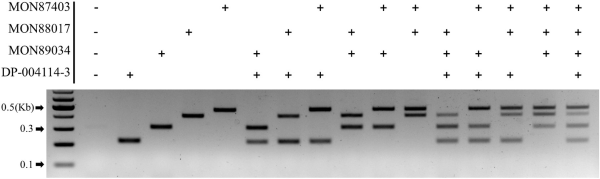
Fig. 4
Limit of Detection (LOD) for LM maize multiplex PCR. The four maize CRMs were successively diluted 1:2 with distilled water, indicating concentrations (100, 50, 25, 12.5, 6.6, 3.1, 1.6, 0.8 ng μL–1). M represents a 100 bp DNA size marker.
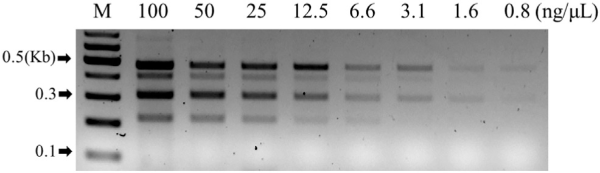
Fig. 5
The application of multiplex PCR in analyzing LM maize monitoring samples from 2021 and 2022 in South Korea. Identification of 17 suspicious LM maize samples from volunteer monitoring, by both multiplex and event-specific PCR. (A) Four CRMs (DP-004114-3, MON89034, MON88017, MON87403) were used as the positive control, and non-LM maize was used as the negative control. Sample names from the LMO monitoring were marked at the top of the agarose gel image (2021 to 2022). The event-specific primers used for each amplification were marked on the right side of the agarose gel image. M represents a 100 bp marker. The maize endogenous gene (Adh1) was used for PCR control. (B) Photos showing the unintentional release of LM maize around transportation routes.
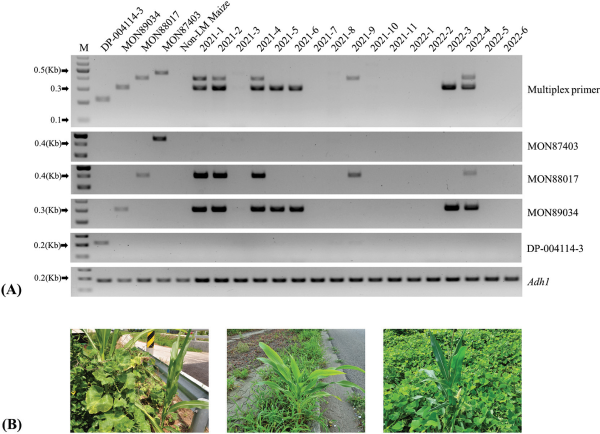
Table 1
Lists of reference maize materials used in this study
No. | Event name | Cat. no. | CRM (or RM) developer | Confidence level (%) | Certified value (g Kg–1) | Uncertainty (g Kg–1) | Type | |||||||
---|---|---|---|---|---|---|---|---|---|---|---|---|---|---|
1 | DP-004114-3 | ERM-BF439b | IRMM | 95 | >986 | N/A | Powder | |||||||
2 | MON89034 | 0906-E2 | AOCS | 95 | ≥996 | –58 ~ +4 | Powder | |||||||
3 | MON88017 | 0406-D2 | AOCS | 95 | ≥990.7 | –72 ~ +9.3 | Powder | |||||||
4 | MON87403 | 0216-A | AOCS | 95 | ≥991 | –31 ~ +9 | Powder | |||||||
5 | Non-LM maize (MON87411, MON87403, MON88017, MON89034, MON87460, MON87427, MON87419) | 0406-A2 | AOCS | 95 | <0.8 | 0.4 | Powder | |||||||
6 | Non-LM maize (GA21, MIR162, MIR604, MZHG0JG) | 0407-A | AOCS | 95 | <1.0 | 0.5 | Powder | |||||||
7 | Non-LM maize (5307) | 0411-C2 | AOCS | 95 | <1.0 | 0.5 | Powder | |||||||
8 | Bt11 | ERM-BF412bk | IRMM | 95 | >970 | N/A | Powder | |||||||
9 | DAS-59122-7 | ERM-BF424d | IRMM | 95 | 98.7 | +5.8 ~ +5.9 | Powder | |||||||
10 | MON87427 | 0512-A2 | AOCS | 95 | >994.8 | –26 ~ +5.2 | Powder | |||||||
11 | MON863 | ERM-BF416d | IRMM | 95 | >98.5 | –2.2 ~ +2.5 | Powder | |||||||
12 | TC1507 | ERM-BF418d | IRMM | 95 | 98.6 | –1.7 ~ +2.0 | Powder | |||||||
13 | VCO-01981-5 | ERM-BF438b | IRMM | 95 | >986 | N/A | Powder | |||||||
14 | DP-098140-6 | ERM-BF427d | IRMM | 95 | 100 | 4 | Powder | |||||||
15 | MON810 | ERM-BF413gk | IRMM | 95 | 99 | 5 | Powder |
Table 2
List of oligonucleotide primers used to develop four LM maize events
Target | Primer name | Primer sequence (5′–3′) | Size (bp) | Reference | ||||
---|---|---|---|---|---|---|---|---|
Alcohol Dehydrogenase 1 (Endogenous Gene) | Adh1_F | CGTGGTTTGCTTGCCCACAG | 179 | Park et al., 2020 | ||||
Adh1_R | CCACTCCGAGACCCTCAGTC | |||||||
DP-004114-3 | DP-004114-3R_V1 | GTCCGCAATGTGTTATTAAGTTGTCTAAGC | 218 | This work | ||||
DP-004114-3R_P5 | GTGAGAACCGTATATCCAAGGTTC | This work | ||||||
MON89034 | MON89034L_V1 | CGACGGATCGTAATTTGTCG | 316 | This work | ||||
MON89034L_P5 | GCTACCCCTGCAGCTTTAA | This work | ||||||
MON88017 | MON88017R_V3 | GTAATCGGCTAATCGCCAACAG | 410 | This work | ||||
MON88017R_P6 | CCGCTCTTTTTGGATGGAC | This work | ||||||
MON87403 | MON87403R_V2 | CGACGGATCGTAATTTGTCG | 464 | This work | ||||
MON87403R_P1 | GGGAAACATAGATGTGGAGGTCAG | This work |